Introduction
The Sustainable Development Goals (SDGs) have set a broad agenda to advance health and achieve equity by 2030. However, promoting healthy lives and well-being for all remains a challenge in resource-limited settings [1]. Many areas in developing countries still suffer from significant socio-economic imbalances and inequalities in access to healthcare resources and quality medical care. Infection prevention and control (IPC) is particularly challenging in rural areas where health resources are often extremely limited [2,4]. Simultaneously, infectious diseases heavily burden population health outcomes and present a formidable challenge for public health. According to the World Health Organization, a high burden of communicable diseases such as malaria, tuberculosis, and HIV/AIDS puts considerable pressure on healthcare systems in developing countries [5]. Access to care in qualified centres is difficult for a large portion of the population in these areas, and as a result, the SDGs for reducing infection-related mortality rates remain unmet (fig 1) [6]. Given these challenges, the availability of healthcare facilities capable of effectively responding to needs in safe and highly sustainable spaces is a key priority for individual and public health, especially in extremely resource-limited rural areas.
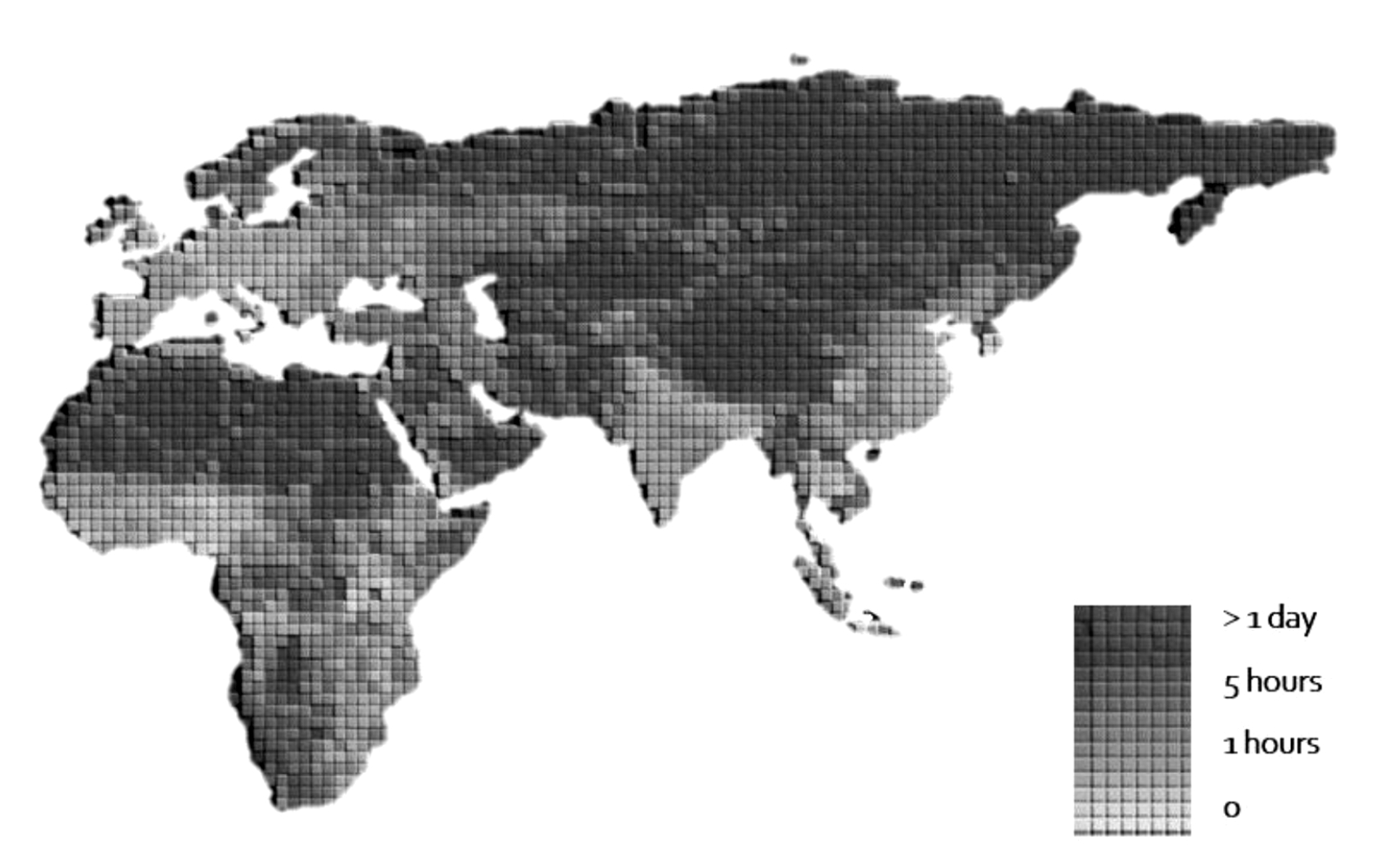
Infection prevention and control in developing countries
IPC represents a fundamental, evidence-based approach aimed at safeguarding patients and healthcare workers from avoidable infections. It influences every aspect of healthcare, including hand hygiene, prevention of surgical site infections, injection safety, antimicrobial resistance, and hospital operations during both emergencies and routine care [4,7-9]. IPC is unique in the realm of patient safety and quality care as it is universally relevant to every healthcare interaction, involving every patient and health worker (fig 2). Achieving effective IPC is a priority in any care programme and requires continuous efforts at all levels of the healthcare system, from policymakers and facility managers to healthcare workers and patients. Supportive IPC programmes are particularly vital in low- and middle-income countries, where healthcare delivery and hygiene standards are often compromised by secondary infections [7-9]. The World Health Organization states that the risk of healthcare-associated infections (HAIs) in developing countries is two to twenty times higher than in industrialised nations. This is due to a combination of factors: limited resources, infrastructural constraints, knowledge gaps, and socio-economic and political factors [10].
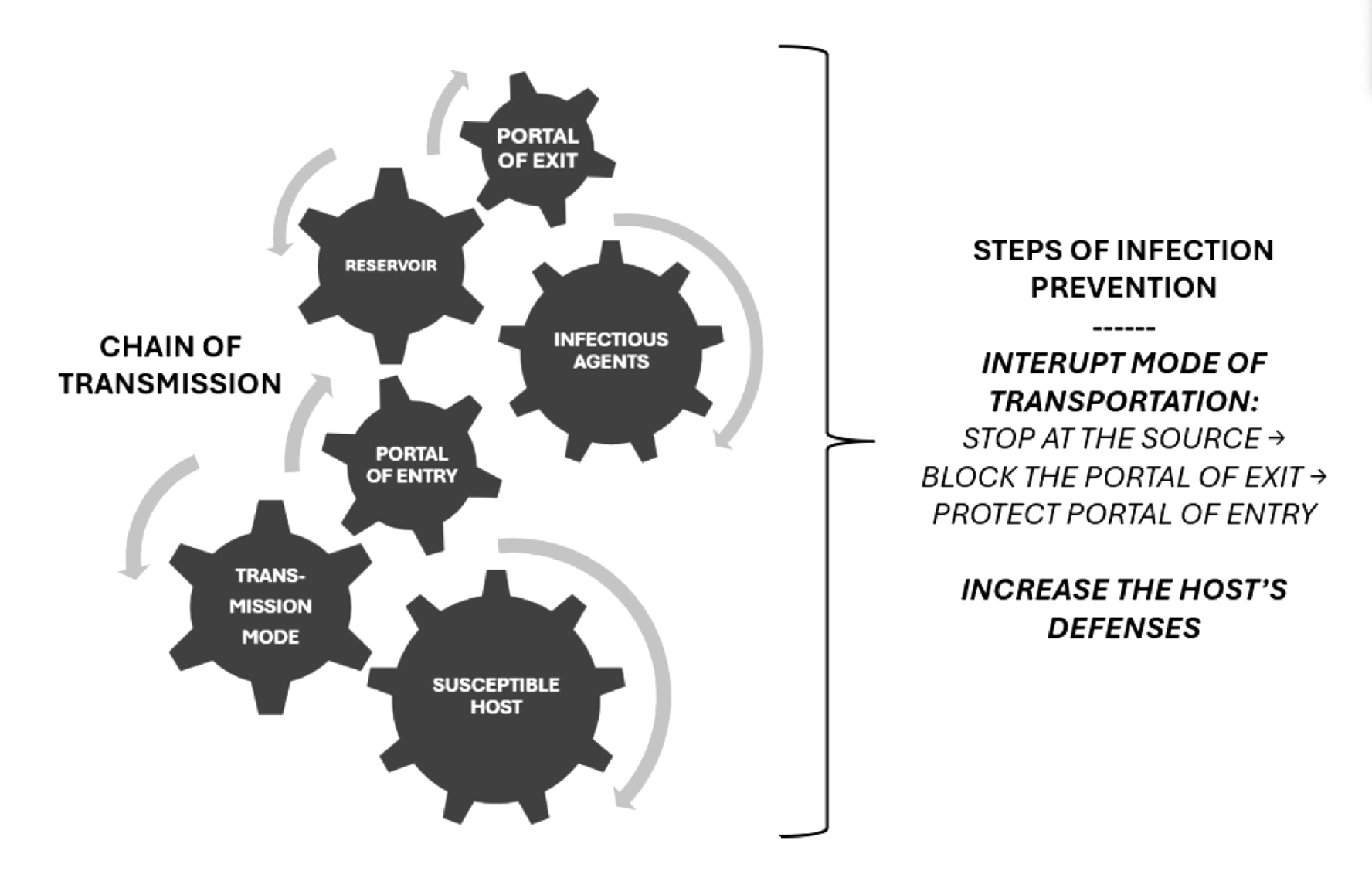
Developing countries often face shortages of essential resources such as clean water, sanitation facilities, personal protective equipment, disinfectants, and trained healthcare workers. Overcrowding in hospitals, inadequate ventilation systems, and poor waste management practices can contribute to the spread of infections. The lack of awareness and training on proper IPC practices among healthcare workers can hinder effective implementation. Moreover, poverty, malnutrition, and limited access to healthcare can increase patient susceptibility to infections.
Improving IPC in developing countries requires a multifaceted approach. Strong political will and investment in healthcare infrastructure, resources, and training programmes are essential. Providing healthcare workers with the necessary resources, training, and support to implement IPC guidelines is crucial. Engaging communities in IPC efforts and promoting health education can lead to sustainable behavior change [11]. Sharing knowledge, best practices, and resources between developed and developing countries can accelerate progress. However, in under-resourced and remote rural settings, where access to high-quality healthcare infrastructure is limited, the challenge of adhering to IPC guidelines is even greater [12]. These areas often face not only high burdens of communicable diseases but also significant geographical and climatic obstacles, combined with unique social, cultural, and spiritual beliefs that collectively contribute to the difficulty of infection control [13]. The presence of small healthcare centres in these regions is crucial for safeguarding public health, yet the challenging environmental conditions prevalent in these areas often lead to minimal adherence to IPC standards. Despite the daunting challenge, there are proven strategies to improve IPC even in resource-limited settings. Key IPC strategies can be summarized in seven main points: hand hygiene, environmental cleaning and disinfection, safe injection practices, waste management, isolation precautions, antimicrobial stewardship, and education and training. Addressing these challenges and implementing effective IPC strategies can create safer healthcare environments and improve health outcomes for all, regardless of where they live [12]. A less explored yet critically important aspect of IPC application is the contribution that targeted hospital space design can make. Architectural resources and strategies can substantially enhance IPC standards even under extreme conditions and at minimal cost. Epidemiologically, the healthcare environment is considered one of the extrinsic factors that affect the infectious agent and the opportunity for exposure. Although the relationship between the healthcare environment and infectious disease prevention and control is gaining recognition, developing the knowledge of efficiently adopting design strategies for infectious disease control remains a significant challenge in developing countries [11,14].
Architectural design contributions to infection prevention and control in extremely resource-limited settings: passive design strategies
Architectural design can significantly contribute to infection prevention and control in healthcare facilities, even in resource-constrained settings. Even small design choices can have a big impact on IPC. By focusing on affordability, sustainability, and local context, architects can make a real difference in creating safer healthcare environments in developing countries. By embracing safe and sustainable design strategies, healthcare facilities in developing countries can move towards a more environmentally responsible, cost-effective, and resilient model of care, ultimately benefiting both present and future generations [15,16]. Finally, by engaging with local communities to understand their needs and practices related to hygiene and healthcare, policy makers and architects can organize and design healthcare facilities culturally appropriate and accepted by the community [17].
Some key strategies are reported in table 1.
IPC Strategies | IPC Concepts | Design Strategies |
Optimizing Natural Ventilation |
Reduces Airborne Pathogens: Good airflow naturally flushes out airborne bacteria and viruses, lowering the risk of transmission.
Cost-Effective: Relies on natural forces, reducing the need for energy-intensive HVAC systems and their maintenance. |
Orientation: Position the building to take advantage of prevailing winds.
Window Placement: High and low windows create a “stack effect,” drawing fresh air in and pushing stale air out. Ventilation Shafts: Vertical shafts can enhance natural airflow through the building. Courtyards: Open spaces within the facility can promote air circulation. |
Optimizing Natural Lighting |
Disinfection: Sunlight has natural germicidal properties, killing some bacteria and viruses on surfaces.
Improves Visibility: Better visibility for cleaning staff to identify and address potential hygiene issues. |
Large Windows: Maximize the amount of natural light entering the building.
Skylights: Introduce sunlight into deeper areas of the facility. Light Wells: Reflect sunlight into interior spaces. Light Shelves: Horizontal surfaces that bounce daylight deeper into rooms. |
Simple and Easy-to-Clean Layouts |
A – Surface Porosity and Cleanability: Prioritize Non-Porous Materials: non-porous materials and solid surfaces are less likely to harbor microorganisms and are easier to clean and disinfect effectively. This reduces the risk of cross-contamination. Minimize Grout Lines and Seams: Seamless surfaces are crucial. Where seams are unavoidable, use antimicrobial sealants and ensure they are properly maintained to prevent germ accumulation.
B – High-Touch Surface Considerations: Frequent Disinfection: Identify high-touch surfaces (door handles, bedrails, light switches, call buttons) and select materials that can withstand frequent disinfection with hospital-grade cleaners without degrading. C – Design for Accessibility and Thorough Cleaning: Easy Access for Cleaning: Ensure adequate space around equipment and furniture for easy cleaning and disinfection. Avoid tight corners or hard-to-reach areas that can be missed during routine cleaning. Wall and Floor Junctions: Use coved baseboards or integral floor-wall transitions to eliminate dirt traps and facilitate thorough cleaning. Note: Surface Properties Compliant With IPC And Job Security VOC Emissions: Low-VOC Materials: Choose materials with low or no volatile organic compound emissions to maintain good indoor air quality and minimize potential health risks. Look for products certified by reputable organizations like Greenguard or FloorScore. Fire Safety: Fire-Resistant Materials: Ensure all materials meet fire safety standards and building codes. Use fire-resistant materials, especially in areas with high fire risks. Sustainability: Environmentally Friendly Materials: Consider using sustainable and recycled materials whenever possible to minimize the environmental impact of construction. Look for products with certifications like LEED or Cradle to Cradle. |
1 – Materials Selection:
Seamless Surfaces: Opt for materials that create smooth, continuous surfaces with minimal grout lines, seams, or crevices where dirt and germs can accumulate. Examples include:
Non-Porous Materials: Choose materials that are resistant to moisture and stains, making them easy to clean and disinfect. Examples include:
Durable Finishes: Select materials with durable finishes that can withstand frequent cleaning and disinfection without degrading. Look for finishes that are:
Antimicrobial Options: Consider using materials with antimicrobial properties, such as copper alloys, which can continuously kill bacteria on contact, providing an added layer of protection. 2 – Design Details: Coved Corners: Design coved (rounded) corners where walls meet floors and ceilings. This eliminates sharp angles that are difficult to clean and can harbor dust and germs. Integrated Features: Integrate sinks, countertops, and backsplashes seamlessly to minimize joints and crevices. Minimal Trim and Molding: Reduce the use of decorative trim and molding, which can collect dust and make cleaning more time-consuming. Recessed Features: Consider recessed soap dispensers, paper towel dispensers, and hand sanitizer dispensers to create a smoother wall surface. Easy-Access Panels: Design access panels for plumbing and electrical systems that are easy to remove and clean. 3 – Additional Considerations: Color Selection: Light-colored surfaces can make it easier to spot dirt and spills, while darker colors may show scratches and wear more easily. Lighting Design: Adequate lighting is essential for effective cleaning. Ensure that all areas are well-lit, paying attention to corners and under furniture. Maintenance Planning: Consider the long-term maintenance requirements of different materials and finishes when making design decisions. |
Decentralized Handwashing Stations |
Strategic Locations: Position stations in high-traffic areas and points of care, such as near room entrances, patient beds, medication preparation areas, and outside restrooms.
Visibility: Ensure stations are easily visible and clearly marked to encourage frequent use. Universal Design: Design stations to be accessible to people of all abilities, considering wheelchair users, those with limited mobility, and children. Splash Reduction: Design sinks and faucets to minimize splashing, which can spread contaminants. |
Integrated Design: Incorporate handwashing stations seamlessly into the overall design of the facility, rather than treating them as afterthoughts.
Space Planning: Allocate sufficient space around stations to allow for comfortable use and to avoid crowding. Lighting: Provide adequate lighting to ensure visibility and promote proper hand hygiene. Signage and Education: Use clear signage to indicate the location of stations and reinforce proper handwashing techniques. Flexibility and Adaptability: Design stations to be modular or adaptable to accommodate future changes in needs or technology. Water Conservation: Explore water-saving features like low-flow faucets and automatic shut-off valves. |
Spatial Separation and Zoning |
A – Risk Stratification and Separation:
Identify Risk Levels: Assess different areas of the facility and categorize them based on the risk of infection transmission (e.g., high-risk areas like operating rooms and isolation rooms, moderate-risk areas like patient wards, low-risk areas like administrative offices). Physical Barriers: Use walls, partitions, doors, and corridors to create physical separation between areas of different risk levels. This helps contain potential outbreaks and prevents the spread of infectious agents. Air Pressure Control: Employ air pressure differentials to control airflow between zones. For example, maintain negative pressure in isolation rooms to prevent airborne pathogens from escaping. B – Zoning for Infection Control: Functional Zones: Divide the facility into distinct zones based on their function (e.g., patient care zones, staff zones, public zones). This helps minimize unnecessary traffic and potential cross-contamination between different user groups. Clean vs. Dirty Workflows: Establish clear pathways for the movement of people, equipment, and materials to separate “clean” and “dirty” workflows. This helps prevent the spread of contaminants from contaminated areas to clean areas. C – Traffic Flow and Wayfinding: Minimize Cross-Traffic: Design circulation routes to minimize the intersection of staff, patients, and visitors from different risk zones. Use separate entrances, hallways, or elevators for different user groups. Clear Signage: Implement clear and intuitive signage to guide people through the facility and reinforce zoning protocols. Visual Cues: Use color-coding, floor markings, or other visual cues to differentiate between zones and guide traffic flow. |
Flexible Design: Incorporate flexible design elements, such as movable partitions or adaptable rooms, to easily modify zoning layouts in response to changing needs or outbreaks.
Visibility and Natural Light: Design spaces with good visibility to promote observation and natural surveillance. Maximize natural light to create a more pleasant and healthier environment. Outdoor Spaces: Integrate outdoor spaces, such as courtyards or gardens, to provide opportunities for fresh air and respite for patients, staff, and visitors. Technology Integration: Consider incorporating technology, such as real-time location systems or digital signage, to monitor and manage traffic flow and communicate zoning protocols effectively. Additional Considerations:
|
Waste Management |
A – Waste Segregation at Source:
Clearly Defined Categories: Implement a color-coded system with clearly labeled containers for different waste streams:
Strategically Placed Containers: Position waste containers in convenient locations near the point of waste generation to encourage proper segregation. B – Waste Minimization: Reduce, Reuse, Recycle: Implement strategies to reduce waste generation, such as using reusable items whenever possible and exploring recycling options for appropriate materials. Inventory Control: Manage medical supplies effectively to minimize expiration and waste. C – Safe Handling and Transport: Closed Containers: Use leak-proof and puncture-resistant containers with lids for all waste streams, especially infectious waste. Minimize Handling: Design workflows to minimize the number of times waste is handled to reduce the risk of exposure. D – Waste Treatment and Disposal: Consider on-site treatment options for certain waste streams, such as autoclaving for infectious waste, to reduce the volume and hazard level before disposal. |
Dedicated Waste Rooms: Designate dedicated, well-ventilated rooms with handwashing stations for waste storage and sorting.
Chutes and Conveyors: Incorporate chutes or automated conveyor systems to safely and efficiently transport waste from upper floors to designated collection areas. Access Control: Limit access to waste storage areas to authorized personnel only. Durable and Easy-to-Clean Surfaces: Use materials for walls, floors, and fixtures that are durable, moisture-resistant, and easy to clean and disinfect. Signage and Training: Provide clear signage to indicate waste segregation procedures and provide comprehensive training to all staff on proper waste management protocols. Additional Considerations:
|
Passive design strategies involve a suite of sustainable architectural techniques that harness natural resources to optimize a building’s performance, comfort, and energy efficiency. Unlike active design systems that depend on mechanical interventions, passive strategies exploit the inherent characteristics of a site to regulate heating, cooling, lighting, and ventilation (fig. 3).
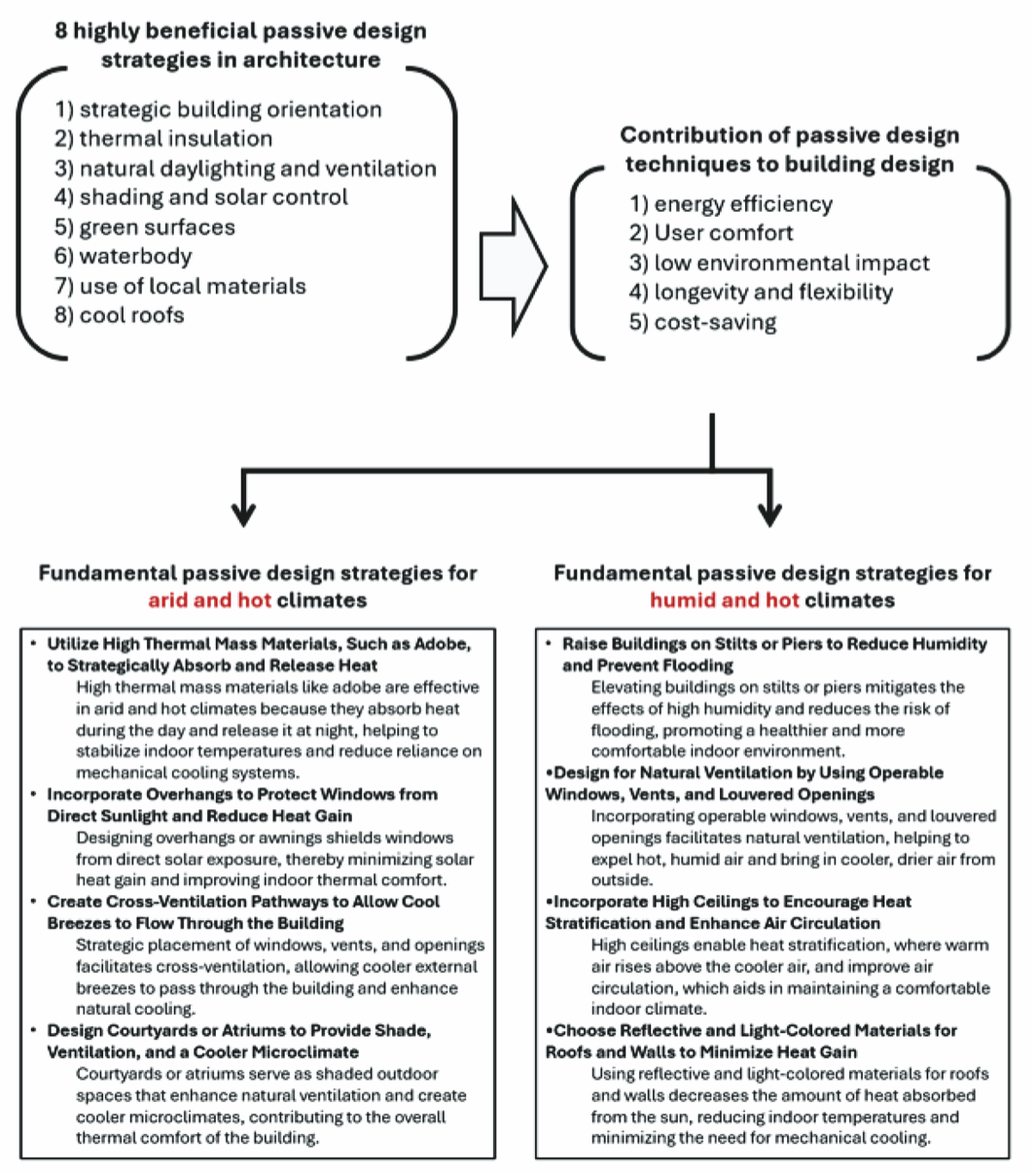
Essential elements such as solar orientation, wind patterns, thermal mass, local material availability, and geological features play a pivotal role in determining the passive strategies for a healthcare facility project [17]. Therefore, it is imperative for architects working on the design of low-resource rural clinics and hospitals in developing countries to explore passive design strategies that minimize environmental and climate impact, and reduce both construction and maintenance costs.
Examples of passive design strategies to enhance natural ventilation and lighting
rom an architectural perspective, natural ventilation leverages differences in air pressure, temperature, and wind to ventilate buildings without the need for mechanical systems [18-23]. This method includes various techniques such as stack ventilation, wind towers, and courtyard effects. Stack ventilation utilizes temperature differences to expel warm air from tall rooms or structures. Wind towers cool incoming air through evaporation before distributing it indoors (fig. 4) [24]. Courtyards facilitate the rise of warm air, which is then replaced by cooler air entering at lower levels (fig. 5) [25]. Optimal natural airflow can be achieved through proper building orientation, strategic openings, cross ventilation, and tall structures. While natural ventilation provides cost-free ventilation, it offers less control over air quality compared to mechanical systems.
Prioritize designs that maximize natural ventilation and sunlight is a key resource for the projects of rural healthcare facilities. As previously reported, good airflow helps to remove airborne pathogens, and sunlight has natural disinfectant properties. Natural ventilation and lighting are valuable and integrated tools for infection control, especially in limited-resource settings where mechanical systems might be expensive or unreliable. By thoughtfully integrating natural ventilation and lighting, architects can create healthcare environments that are healthier, more sustainable, and better suited for resource-constrained settings. This can be achieved through strategically placed windows, courtyards, and building orientation [6-7]. Key architectural considerations are that:
- design should be tailored to the local climate to ensure comfort and effectiveness;
- cultural preferences and practices related to ventilation and light should be strictly considered for the acceptability of the healthcare users;
- natural ventilation should be balanced with infection control measures, such as appropriate spatial separation, to prevent cross-contamination.
Integrating natural ventilation and lighting in healthcare facilities in developing countries contributes to a more sustainable and resilient healthcare infrastructure in several ways. Firstly, this architectonic strategy reduces environmental impact by decreasing reliance on energy-intensive HVAC systems and artificial lighting, natural alternatives significantly lower a facility’s carbon footprint and operational costs. Natural ventilation and lighting systems are less susceptible to power outages or mechanical failures, making them more resilient in the face of natural disasters or infrastructure challenges often faced in developing countries. The reduced reliance on mechanical systems translates to significant cost savings on energy bills and maintenance, freeing up resources for other critical healthcare needs. This is particularly impactful in regions with unreliable electricity grids or limited resources. Moreover, by improving indoor air quality and providing natural light, these strategies can contribute to a healthier healing environment, potentially reducing the spread of infections and improving patient recovery times [8]. Natural ventilation and lighting can create a more comfortable and pleasant environment for patients, staff, and visitors, potentially reducing stress and improving overall well-being. Finally, utilizing readily available resources like sunlight and wind can empower local communities to participate in the construction and maintenance of their healthcare facilities, fostering a sense of ownership and sustainability.
Perspectives: strategies to achieve net zero healthcare facilities in developing countries
The drive towards sustainability in the built environment has increasingly centered on achieving net-zero energy consumption, especially in the context of workplace design [25]. The net-zero workplace design framework goes beyond the basic goal of environmental impact reduction. It offers a holistic approach that addresses both environmental sustainability and the well-being of occupants. This design philosophy incorporates natural ventilation, maximizes daylight exposure, and fosters the creation of inspiring work environments, all of which play a vital role in enhancing the quality of life for patients and workers [26-28]. It is important to understand that achieving a net-zero workplace involves a comprehensive approach that includes several critical components. At the core of this endeavor is the principle of energy efficiency, which focuses on reducing energy consumption through the implementation of advanced systems and practices designed to minimize waste and optimize the use of resources. This involves adopting measures such as high-efficiency lighting, advanced HVAC systems, and energy-saving building designs. In addition to enhancing energy efficiency, another fundamental aspect of creating a net-zero workplace is the incorporation of renewable energy sources. This involves integrating clean energy solutions like solar panels, wind turbines, or geothermal systems to meet the workplace’s energy demands. By relying on these renewable sources, businesses can significantly reduce their dependence on fossil fuels and move towards a more sustainable energy future [27]. However, achieving net-zero energy consumption also requires addressing any remaining carbon emissions through carbon offsetting. This final step involves compensating for residual emissions by engaging in activities such as tree planting or investing in carbon offset projects. These efforts help to balance out the carbon footprint of the workplace, thereby contributing to the broader goal of environmental sustainability. Together, these components – energy efficiency, renewable energy, and carbon offsetting – form the foundation of a net-zero workplace strategy. Each element plays a crucial role in not only reducing the environmental impact of workplace operations but also in fostering a more sustainable and responsible approach to energy use and carbon management [26-28]. To achieve a fair and effective transition to net-zero healthcare, strategies must be progressive rather than uniform, addressing population dynamics and essential needs, and ensuring that the most significant polluters reach net-zero emissions sooner to create opportunities for others. Accelerating the net-zero healthcare agenda can not only reduce the climate impact of healthcare practices but also drive the societal transformation essential for meeting the Paris Agreement’s climate objectives. In this decisive decade for climate action, the health sector must play an active and leading role.
Conclusions
One of the key challenges for scientists is health-care innovation in developing nations. Creating a low-cost innovation to address a global health problem is not easy. Scaling it up, securing financing, ensuring distribution, achieving cultural acceptance, and actual utilization are even more challenging. Success depends on understanding the conditions and resources of the local community. Working side by side, integrating diverse disciplines and expertise, even when they seem vastly different and distant, is the only path to achieving widespread and global health.